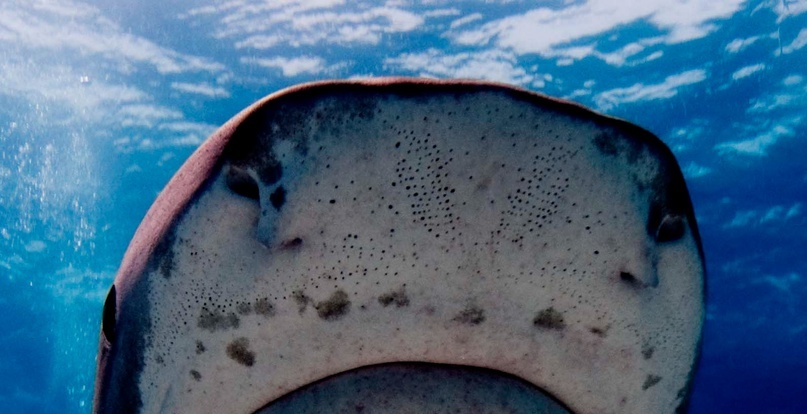
Like other animals, sharks have all five senses - sight, smell, touch, taste, tactility. They have one more additional 6th sense, which distinguishes them from all the others - electroreception.
What is electroreception and how does it work?
This is a biological ability to perceive electromagnetic impulses. An ancient sense that developed independently in the animal kingdom in several groups, including jawless (lampreys), cartilaginous (chimaeras, sharks, rays) and bony fish (lungfish, coelacanths, polypterids, cartilaginous and bony), some amphibians and mammals.
Discovered this ability in sharks - In 1971, Adrianus "Ad" Kalmijn, he published a fascinating article proving that sharks and rays have an additional sense that perceives electromagnetic waves.
What is electroreception and how does it work?
This is a biological ability to perceive electromagnetic impulses. An ancient sense that developed independently in the animal kingdom in several groups, including jawless (lampreys), cartilaginous (chimaeras, sharks, rays) and bony fish (lungfish, coelacanths, polypterids, cartilaginous and bony), some amphibians and mammals.
Discovered this ability in sharks - In 1971, Adrianus "Ad" Kalmijn, he published a fascinating article proving that sharks and rays have an additional sense that perceives electromagnetic waves.
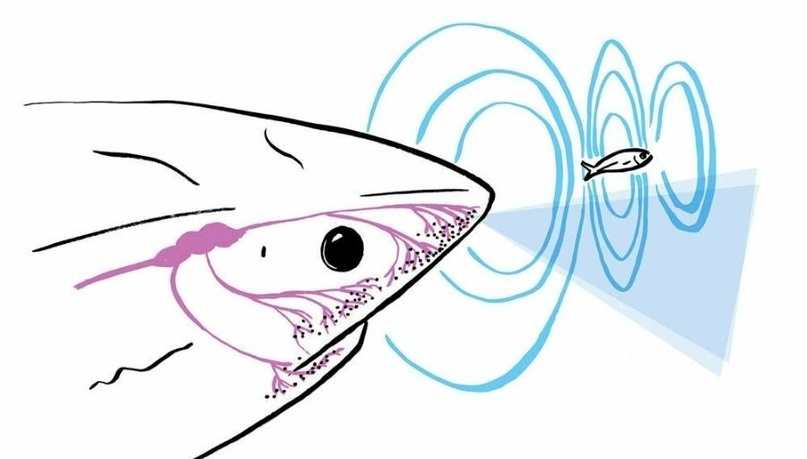
Schematic diagram of the principle of operation of electroreception in sharks
How was it discovered?
Italian ichthyologist Stefano Lorenzini formally described the anatomy of these organs in the 17th century. They are a system of tiny pores located all over the head of the fish. But what no one knew was what these pores were used for. There was a lot of speculation, but no proof.
A key breakthrough came in 1935, when physiologist Sven Dijkgraaf of Utrecht University in the Netherlands was studying sharks for another project when he noticed that a captive shark reacted when approached by a rusty wire in his aquarium. The shark retreated from the wire after being approached, recoiling in apparent disgust or pain-like reaction, even though the fish had never touched it. And this flight response occurred even when the shark was blindfolded and could not see the wire. Clearly, the wire was not providing a visual stimulus that the shark could detect. In the 1960s, chemist Royce Murray of the University of North Carolina at Chapel Hill documented that the sensory cells in the ampullae of Lorenzini responded to electromagnetic fields. But this work was done in the lab only with the ampullae organs, not with a live, swimming shark. Adrianus "Ad" Kalmijn finally demonstrated this ability in 1971, when he conducted a series of clever experiments in which he placed live prey in protective agar sheaths so that the sharks could not see, hear, or smell them, but could still sense their bioelectric fields. In doing so, he answered the questions, "Are there electric fields in the natural habitats of sharks and rays that can be detected by these animals?" and "If so, do sharks and rays actively use these fields?"
Italian ichthyologist Stefano Lorenzini formally described the anatomy of these organs in the 17th century. They are a system of tiny pores located all over the head of the fish. But what no one knew was what these pores were used for. There was a lot of speculation, but no proof.
A key breakthrough came in 1935, when physiologist Sven Dijkgraaf of Utrecht University in the Netherlands was studying sharks for another project when he noticed that a captive shark reacted when approached by a rusty wire in his aquarium. The shark retreated from the wire after being approached, recoiling in apparent disgust or pain-like reaction, even though the fish had never touched it. And this flight response occurred even when the shark was blindfolded and could not see the wire. Clearly, the wire was not providing a visual stimulus that the shark could detect. In the 1960s, chemist Royce Murray of the University of North Carolina at Chapel Hill documented that the sensory cells in the ampullae of Lorenzini responded to electromagnetic fields. But this work was done in the lab only with the ampullae organs, not with a live, swimming shark. Adrianus "Ad" Kalmijn finally demonstrated this ability in 1971, when he conducted a series of clever experiments in which he placed live prey in protective agar sheaths so that the sharks could not see, hear, or smell them, but could still sense their bioelectric fields. In doing so, he answered the questions, "Are there electric fields in the natural habitats of sharks and rays that can be detected by these animals?" and "If so, do sharks and rays actively use these fields?"
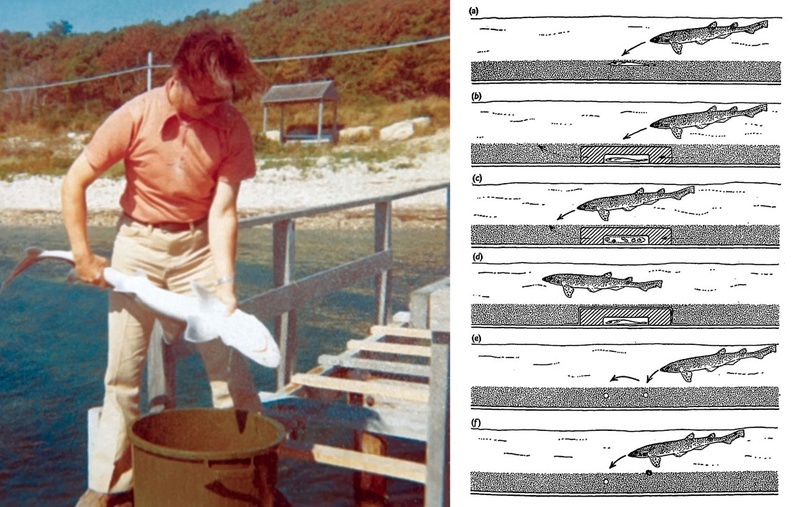
In the photo: Adrianus Kalmijn holding a common catshark (Scyliorhinus canicula). And also a diagram of the experiment conducted from his article on shark electroreception
How do they do it?
All sharks have special organs for detecting electromagnetic waves - electroreceptors (ampullae of Lorenzini). They are jelly-filled tubes that open on the surface of the shark's skin. Inside, each tube ends in a bulb known as an ampulla. If you remove the skin from the head of a shark, you can see hundreds of these bulbs. The jelly in the tube is highly conductive, which allows the electrical potential to be transmitted from the pore to the ampulla at the base of the tube. The potential difference across the membrane lining each ampulla activates nerves that send signals to the brain. In addition, as it turns out, this system is connected to the lateral line organ. Thus, sharks simultaneously sense electromagnetic radiation and vibrations of water molecules.
Sharks are helped in this by nature itself. Seawater is an ion-rich medium that conducts electric fields moderately well. Seawater moving over the magnetic field lines of our planet creates a weak but richly textured electrical "map" of the immediate surroundings. The shark's body contains a rich broth of electrically charged biomolecules called electrolytes, which allow cells to communicate with each other.
The amount of muscle contraction affects the magnitude of the electric field. The more muscles contract, the greater the field, and vice versa. In addition, the intensity of the electric fields changes when the animal is injured.
For example, crustaceans can generate a voltage of 50.0 mV, measured with a sensor electrode placed 1 mm from the animal's surface. The same crustaceans have been shown to generate a much higher voltage of 1250.0 mV when injured. It was back in 1947 that biologist Burr originally identified the presence of these bioelectric fields near marine animals.
In 1998, graduate student Steve Kaijura demonstrated that newborn shovelnose sharks (Sphyrna tiburo) can detect electric fields of less than 1 nanovolt per square centimeter. This is equivalent to the electric field of a flashlight battery connected to electrodes spaced about 10,000 miles (16,000 kilometers) apart in the ocean. This incredible electrical sensitivity is more than five million times greater than anything you or I could sense, and is certainly the most acute in the Animal Kingdom.
Coupled with this, sharks sense not only the muscles, but the heart and brain activity of potential prey. Especially when injured and stressed, since this generates much more voltage in the nervous system. So sharks do, in a sense, feel fear, but on a physical level.
All sharks have special organs for detecting electromagnetic waves - electroreceptors (ampullae of Lorenzini). They are jelly-filled tubes that open on the surface of the shark's skin. Inside, each tube ends in a bulb known as an ampulla. If you remove the skin from the head of a shark, you can see hundreds of these bulbs. The jelly in the tube is highly conductive, which allows the electrical potential to be transmitted from the pore to the ampulla at the base of the tube. The potential difference across the membrane lining each ampulla activates nerves that send signals to the brain. In addition, as it turns out, this system is connected to the lateral line organ. Thus, sharks simultaneously sense electromagnetic radiation and vibrations of water molecules.
Sharks are helped in this by nature itself. Seawater is an ion-rich medium that conducts electric fields moderately well. Seawater moving over the magnetic field lines of our planet creates a weak but richly textured electrical "map" of the immediate surroundings. The shark's body contains a rich broth of electrically charged biomolecules called electrolytes, which allow cells to communicate with each other.
The amount of muscle contraction affects the magnitude of the electric field. The more muscles contract, the greater the field, and vice versa. In addition, the intensity of the electric fields changes when the animal is injured.
For example, crustaceans can generate a voltage of 50.0 mV, measured with a sensor electrode placed 1 mm from the animal's surface. The same crustaceans have been shown to generate a much higher voltage of 1250.0 mV when injured. It was back in 1947 that biologist Burr originally identified the presence of these bioelectric fields near marine animals.
In 1998, graduate student Steve Kaijura demonstrated that newborn shovelnose sharks (Sphyrna tiburo) can detect electric fields of less than 1 nanovolt per square centimeter. This is equivalent to the electric field of a flashlight battery connected to electrodes spaced about 10,000 miles (16,000 kilometers) apart in the ocean. This incredible electrical sensitivity is more than five million times greater than anything you or I could sense, and is certainly the most acute in the Animal Kingdom.
Coupled with this, sharks sense not only the muscles, but the heart and brain activity of potential prey. Especially when injured and stressed, since this generates much more voltage in the nervous system. So sharks do, in a sense, feel fear, but on a physical level.